Fundamental quantum physics
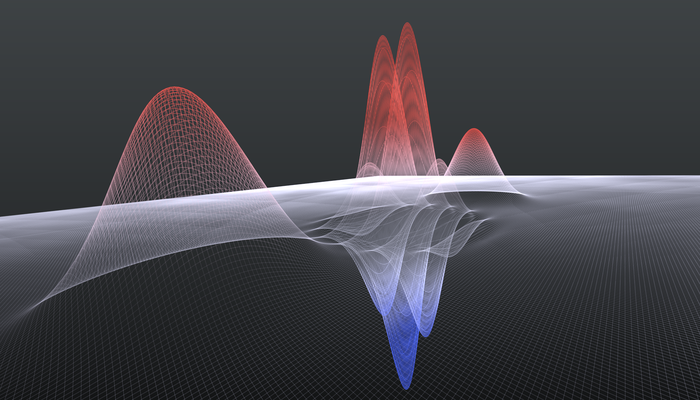
At the quantum scale, the laws of nature deviate drastically from the physics we experience in everyday life. Classical notions of cause and effect break down, and the behaviour of light and matter is governed by intriguing phenomena such as superposition, which makes it impossible to ascribe physical properties to a system (such as whether an atom is here or there) until it is measured, and entanglement, which allows separate physical systems to be correlated stronger than anything possible classically, even across great distances. The physics in this regime is described by the theory of quantum mechanics and opens novel possibilities for secure information processing, communication, and sensing with precision beyond what is possible with classical techniques.
At QPIT, we are excited to expand our understanding of the foundations of quantum mechanics and the fundamental properties of quantum systems. New insights into fundamental quantum physics strengthens our basic understanding of nature and is key to the development and realisation of new applications in quantum-enhanced sensing and quantum information processing, which we also explore at QPIT.
Quantum light, diamonds, and mechanics
In our laboratories, we employ quantum states of light, optically active impurities in diamond (known as color centers), and microscopic mechanical oscillators. All these systems can be coupled together and quantum-mechanically correlated and we strive to achieve an extremely high level of control, employing lasers, high-quality optics, cryogenic cooling, and highly efficient photo detectors.
We characterise the fundamental optical and mechanical properties of our diamonds and mechanical oscillators. In order to develop highly stable and controllable systems with well-preserved quantum properties, for sensing and fundamental physics, we investigate loss mechanisms and decoherence effects, and iterate our designs. To this end, we combine experimental techniques with advanced theoretical modelling of our systems.
We also generate and characterise highly non-classical states of light in both continuous and discrete degrees of freedom of the electromagnetic field. In particular, we create strongly squeezed states, in which the uncertainty of one field quadrature is reduced much below the limit given by the Heisenberg uncertainty relation, as well as highly multipartite entangled states, which display quantum correlations across thousands of separate light pules.
Macroscopic quantum states
While quantum phenomena were first observed at the very small scales of atoms and molecules, there is as yet no known fundamental reason that quantum mechanics should not apply at much larger, and even macroscopic, scales.
QPIT is home to bigQ - Center for Macroscopic Quantum States, a Danish National Research Foundation Center of Excellence dedicated to pushing the boundary between classical and quantum physics by generating and controlling quantum states at increasingly large scales.
We explore macroscopic quantum phenomena by controllably bringing mechanical oscillators, containing vast numbers of atoms, into the quantum regime, and by the generation of quantum states of light entangled across many modes and containing large numbers of photons. Such states can then further be exploited e.g. for ultra-precise sensing and for quantum computing.
Quantum thermodynamics
Another boundary where macroscopic, classical and quantum physics meet is quantum thermodynamics. Classical thermodynamics is a powerful physical theory, which makes profound, universal statements about nature, based on the study of simple, heat-based machines, such as steam engines.
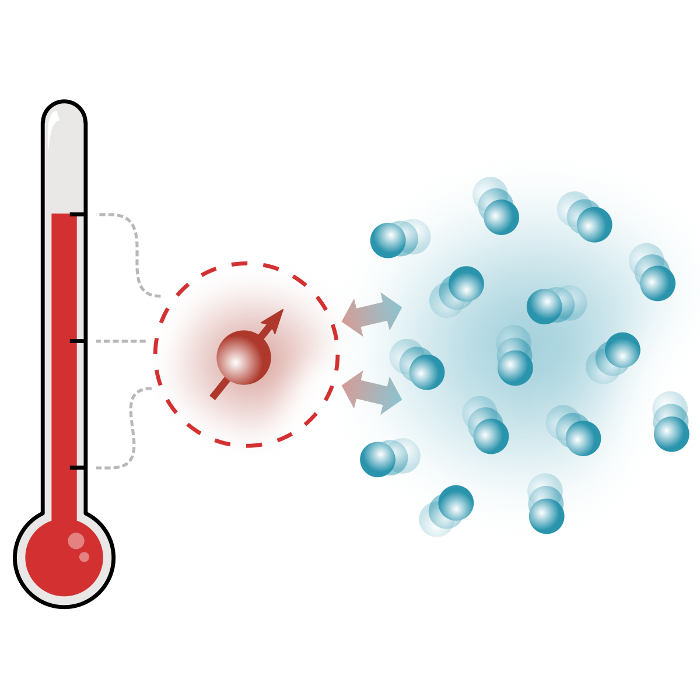
In the quantum regime, however, subtle modifications to the thermodynamic laws of nature are necessary. The performance limits of quantum thermal machines can be different from their classical counterparts. At the same time, it becomes possible to design 'steam engines' that produce not work but resources for quantum information processing, such as entanglement.
At QPIT, we take part in the exciting, ongoing development to expand our understanding of quantum thermodynamics by exploring the theory of quantum thermal machines and entanglement engines. We also explore the fundamental limitations to measuring temperature in quantum systems, connecting to our other activities in quantum sensing.
Quantum nonlocality
Quantum nonlocality is at the heart of quantum theory. The outcomes of certain measurements on entangled quantum systems cannot be explained by any possible theory which obeys classical notions of cause and effect. The structure of such nonlocal distributions is intricately linked to the fundamental structure of the theory of quantum mechanics. More recently, it has also been recognised that demonstrations of nonlocality can be explored for ultra-secure information processing. It enables security to be established in a setting of minimal trust, known as device independent, where devices can be treated as black boxes.
At QPIT, we contribute to development of the theory of nonlocality, and to the design and implementation of new cryptographic and random-number-generation protocols in device-independent and semi-device-independent settings.